Written by:
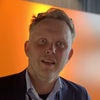
Senior Principal Scientist, Medicinal Chemistry, Early Cardiovascular, Renal and Metabolism, BioPharmaceuticals R&D, AstraZeneca
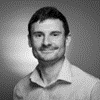
Director, Global High-Throughput Experimentation, Pharmaceutical Sciences, BioPharmaceuticals R&D, AstraZeneca
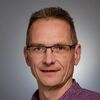
Principal Scientist Chemical Development, Global Operations, AstraZeneca
Sustainability is a critical global issue for people, society and the planet. In the pharmaceutical industry, we have a responsibility to develop medicines that maintain the highest medical efficacy and safety standards to benefit patients, while ensuring a healthier future by minimising the environmental impact on society and the planet. As our technologies and healthcare systems evolve, we have an obligation to continually reduce the use of energy, water, materials, waste, and pollution associated with designing and manufacturing medicines, and delivering them to patients. To drive positive change beyond the impact of our medicines, we are embedding Green Chemistry across our laboratories and manufacturing to enable a healthier, more sustainable future.
What is Green Chemistry?
Green Chemistry, or ‘sustainable chemistry’, is the design of chemical products and processes that reduce or eliminate the use and generation of hazardous substances, use waste disposal as a last resort, and improve energy efficiency across research, development and manufacturing. Green Chemistry techniques have gained global attention for their potential to innovate and advance drug discovery and development while achieving ambitious sustainability goals.
Learn more about how we are implementing Green Chemistry across product design, production, and beyond.
The 12 principles of Green Chemistry
Green Chemistry is based on an externally developed framework of 12 principles designed to maximize efficiencies and minimize hazardous effects on human health and the environment. The framework encourages chemists to use greener chemicals, processes or products that increase the efficiency of experiments, and to find new ways to reduce waste, conserve energy and eliminate the use of hazardous substances.
Green Chemistry and sustainable drug design
Our discovery efforts internally and through external collaborations with leading research institutes and universities have helped us develop new tools and processes that ensure sustainable drug design without compromising safety or effectiveness.
Increasing efficiency in drug discovery through late-stage molecule modification
Late-stage functionalisation is a new way to modify molecules late in their synthesis – helping chemists find ‘shortcuts’ to discovering the next wave of innovative medicines. This can reduce reaction times and the number of resource-intensive reaction steps, and thereby allow our chemists to generate molecular diversity more quickly and more sustainably. We have already used late-stage functionalisation to make over 50 different drug-like molecules, and we are leveraging this technique to develop our next-generation therapeutics.
Creating ‘magic’ with late-stage functionalization
Sometimes, adding even a single methyl group – a single carbon bonded to a hydrogen – can dramatically change the function of a compound; medicinal chemists refer to this as the ‘magic methyl effect’. In 2020, we published a ground-breaking study in Nature Chemistry, in which we were able to add a ‘magic methyl’ group to drugs in just a single step.
Inspired by this result, we collaborated with world-leading academic groups. In our recent study published in Nature Communications, we developed a novel, flexible strategy to selectively add different functional groups to drug compounds at precise molecular locations. Adding functional groups in this way means we can generate many variations of candidate drug molecules in a single step, without having to start from scratch each time.
Innovating the synthesis of PROTACs:
PROteolysis TArgeting Chimeras (PROTACs) are a promising new treatment modality for numerous diseases including cancers and chronic diseases – but they are technically difficult to synthesize. We recently developed a novel method, published in Nature Communications, which uses late-stage functionalization to selectively turn active pharmaceutical ingredients into PROTACs in just a single step, enabling faster and more efficient synthesis of these complex compounds.
Miniaturizing chemical reactions
In collaboration with Stockholm University, Sweden, we are ‘miniaturizing’ chemical reactions – using as little as 1mg of starting material (think: pinch of salt) to perform thousands of reactions. This allows us to explore novel chemistry because we can perform several thousand times more reactions compared to standard techniques with the same amount of material. This method, published in JACS Au, is a new sustainable way to explore a much larger range of drug-like molecules.
Harnessing artificial intelligence and machine learning
Machine learning can help predict and allow us to optimise chemical reactions to make processes more efficient and sustainable. By analysing large datasets of chemical reactions, machine learning algorithms can help our chemists identify patterns, predict reaction outcomes, and optimise reaction conditions. This can help reduce waste, energy consumption, and unwanted byproducts.
Research recently published in the Journal of The American Chemical Society demonstrates our use of a machine learning model that forecasts where a particular chemical reaction – borylation – will occur within complex molecules. This model outperforms previous methods, allowing us to streamline drug development while simultaneously contributing to environmental sustainability.1
Innovating sustainable catalysts
The right catalyst is crucial to greener chemical reactions. Catalysts can speed up reactions, enable new chemistries (that would otherwise be impossible), and crucially, reduce the number of steps required to make an active pharmaceutical ingredient. The right catalyst can also limit the use of environmentally harmful reagents and our reliance on precious metals. Across our research, development and manufacturing, we use a number of innovative catalysts, from light and electricity to biocatalysts and sustainable metals.
Photocatalysis
We recently developed a photocatalyzed reaction that removes several stages from the manufacturing process for a late-stage cancer medicine, leading to a more efficient manufacture with less waste
Visible-light-mediated catalysis has emerged as a powerful tool in organic chemistry, enabling the synthesis of crucial building blocks for drug design under low temperatures. This technology also allows rapid testing of diverse compounds, employs safer reagents, and opens new synthetic pathways for more efficient chemical synthesis to create novel compounds, including one currently in late-stage development.2
In an industry-academia collaboration with the Leonori Group at RWTH Aachen University, we have developed new photocatalysis methods to synthesize various chemical compounds during drug discovery.3,4
Electrocatalysis
Electrocatalysis enables access to unique reaction pathways under mild conditions while also replacing harmful chemical reagents
Electrocatalysis uses electricity to drive chemical reactions, offering an efficient and sustainable route to organic synthesis.
In a collaborative study published in Nature Communications with the Göttingen University and the German Centre for Cardiovascular Research, we applied electrocatalysis to selectively attach a carbon unit that is ready to make libraries of new druglike compounds. This innovative process has the potential to sustainably diversify and streamline the production of candidate molecules.5
Biocatalysis
Biocatalysts stand apart from conventional catalysts, often achieving in a single synthetic step what can take many steps using traditional methods
Biocatalysts are proteins that accelerate chemical reactions. Using biocatalysts can offer more streamlined routes to complex drug molecules compared with traditional synthesis. Advances in computational enzyme design combined with machine learning are expanding the range of biocatalysts available for a wider spectrum of chemical reactions, transforming sustainable synthesis in drug discovery and beyond.
Sustainable metal catalysis
Replacing palladium with nickel-based catalysts in borylation reactions has led to reductions of more than 75% in CO2 emissions, freshwater use, and waste generation
Palladium is used to catalyse crucial chemical reactions; however, platinum group metals (PGMs) such as palladium are expensive and environmentally damaging to source. We are therefore working to identify and optimise catalysts that use the more abundant nickel in two widely used chemical reactions – borylation, and the Suzuki reaction.
No small (scale) feat: reducing waste in manufacturing and product sustainability
We are constantly reducing the quantities of materials used in laboratory experiments by using miniaturization, high-throughput technologies and computational approaches. However, once an active pharmaceutical ingredient (API) leaves the research laboratory and is developed into an approved medicine that requires large-scale manufacturing, the required quantities increase significantly (from kgs to multi-tonnes) – as does the associated waste. This manufacturing waste can be key contributor to our carbon footprint, so extending Green Chemistry principles to our manufacturing is important to delivering our sustainability targets and carbon ambitions.
In line with the Twelve Principles of Green Chemistry, finding shorter sequences of reactions to create an API from simple starting materials is a major focus for our development chemists. Typically, there are multiple possible routes to synthesis – and the more complex API, the more potential routes exist.
One way our chemists assess the available synthesis routes is by using process mass intensity (PMI), a simple sum of the quantity of input materials required to produce a single kg of API. Many of these input materials, such as solvents, catalysts and reagents do not end up in the API but rather, become waste, so minimizing PMI also helps reduce how much waste we produce.
We recently developed a novel method to predict the PMI of all possible routes for a sequence of reactions – without doing any experimentation; this means we can save time and resources not only in the final manufacturing process, but also while optimizing these processes during development.6 In addition, we are exploring other ways to reduce manufacturing waste both in the development phase and post launch, including intensifying our processes, reducing our dependence on solvents, recovering and reusing solvents, and switching to new renewable materials.
Join Us: Working together to deliver sustainable health for future generations
We are focused on accelerating the delivery of life-changing medicines while embedding sustainability into everything we do. By reducing our carbon footprint, our use of Green Chemistry helps us achieve our sustainability goals and demonstrates how strategies that support a healthier planet can also improve human health and advance science.
We welcome committed, talented prospective employees to join us as we create a healthy future for people, society and the planet.